1. Introduction
Climate change is currently a serious problem that threatens human health and has affected the transmission and spread of a wide range of diseases, especially diseases transmitted through vectors [
1]. Climate change refers to long-term statistical changes in weather factors, such as temperature, precipitation, humidity, and sunlight. Evidence shows that climate change significantly affects the public health. The life cycle and transmission of most infectious agents are also effectively related to climate [
2-
4].
Vectors act as intermediate hosts in the life cycle of pathogens and play a role in transmission to living organisms, called hosts. The change in the geographical location and population of vector insects is related to the pattern and climate changes. Accordingly, climate changes may lead to alterations in the scope, period, and severity of infectious diseases by impacting disease vectors [
2].
Vector arthropods are among the ectothermic animals; therefore, they are sensitive to climatic factors. Climate affects the survival and reproduction of vectors, which in turn influences the suitability of the habitat, distribution, frequency, intensity, and temporal pattern of vector activity (especially the biting rate) throughout the year, as well as the growth, survival, and reproduction of pathogens in vectors. However, besides the climate, many other factors affect the distribution of vectors, including the destruction of habitats, land use change, use of poisons and pesticides, and host density [
5, 6].
Temperature affects the spatial and temporal distribution of disease vectors so with the increase in temperature, insects in low-latitude regions may appear in new habitats in middle and high latitudes, and as a result, geographical spread or disease change in high latitudes can be observed. Recent studies have shown that some vector-borne infectious diseases, including malaria, African trypanosomiasis, Lyme disease, tick-borne encephalitis, yellow fever, plague, and dengue fever have spread to wider areas [
7].
The Anopheles mosquito carrying malaria is mostly confined to areas with an average temperature of 16°C; however, as the planet warms, its range can expand dramatically northward. Also, warmer temperatures accelerate the life cycle of disease-carrying insects and lead them to feed more; as a result, more people are significantly infected in warmer climates [
8]. Studies show that the increase in the mortality from vector-borne diseases, including malaria and dengue fever, is because of an increase of nearly 1°C in the global average temperature [
9]. Accordingly, climate change creates conditions that can lead to the spread of infectious diseases.
Following the expansion of vector habitats, including mosquitoes, ticks, and field insects, many of these diseases have spread to high-latitude areas. The issue of habitat change has been observed even in snails, the intermediate host of parasites, for example, in China, with the increase in winter temperatures. In addition, the geographic distribution of the snail Oncomelania hupensis, the intermediate host of Schistosoma japonicum, to new areas in North China has expanded [
10].
The prevalence and occurrence of infectious diseases is affected by climate change. Among these diseases, vector-borne diseases, especially the parasitic diseases caused by mosquitoes are among the diseases sensitive to the climate so the scope and geographical range of several vector-borne diseases and their vectors have changed because of the warming of the air in high areas. Climate change refers to the long-term changes in weather conditions as well as changes in the pattern of severe weather events that may lead to threats to human health and have a double impact on existing health problems [
11].
Numerous studies have been conducted on the impact of climate change and infectious diseases [
3,
12]; however, studies that emphasize the impact of climate on parasitic vector-borne diseases are limited and most studies have focused on diseases, such as malaria [
13] and leishmaniasis [
14, 15]. Hence, this study aims to determine the effect of climate change on infectious diseases with an emphasis on vector-borne parasitic diseases by examining scientific evidence.
2. Materials and Methods
In this review research, a comprehensive search and review of articles were conducted in databases and international scientific sources, including Google Scholar, PubMed, Scopus, and Web of Science based on the title and abstract. In this study, keywords were used with two approaches. In the first approach, the keywords “climate change,” “climate diversity,” “global warming,” “climate changes,” and “severe weather events” were searched, while in the second approach, the keywords “parasitic disease,” “vector born disease,” and “insect born disease” were used. The medical subject heading (MeSH) terms were also used for the search strategy. In this study, articles published from 2009 to 2022 were used to update and obtain the latest information in this field.
In the search for articles, all studies conducted in this field, including descriptive studies, clinical trials, systematic reviews, and all types of review studies related to the purpose of the research were extracted and analyzed. Articles without enough evidence, such as conference reports and lectures were excluded. Of all the sources that were studied in line with the study goal, articles whose full text was accessible were selected.
Also, in this study, based on the review of related publications during the last decade, the terms used to review the literature were tried to reflect 3 aspects, namely climatic and environmental factors and variables, components related to the vector of the disease, as well as infectious diseases with a focus on parasitic vector-borne diseases.
3. Results
The effect of climate on the growth rate, mortality, and reproduction of vectors
As previously mentioned, disease-carrying arthropods and insects are among the organisms sensitive to climate change, and the diseases caused by these vectors are also affected by climate, weather, and environmental conditions, especially parasitic diseases caused by mosquitoes are among climate-sensitive diseases (
Table 1).
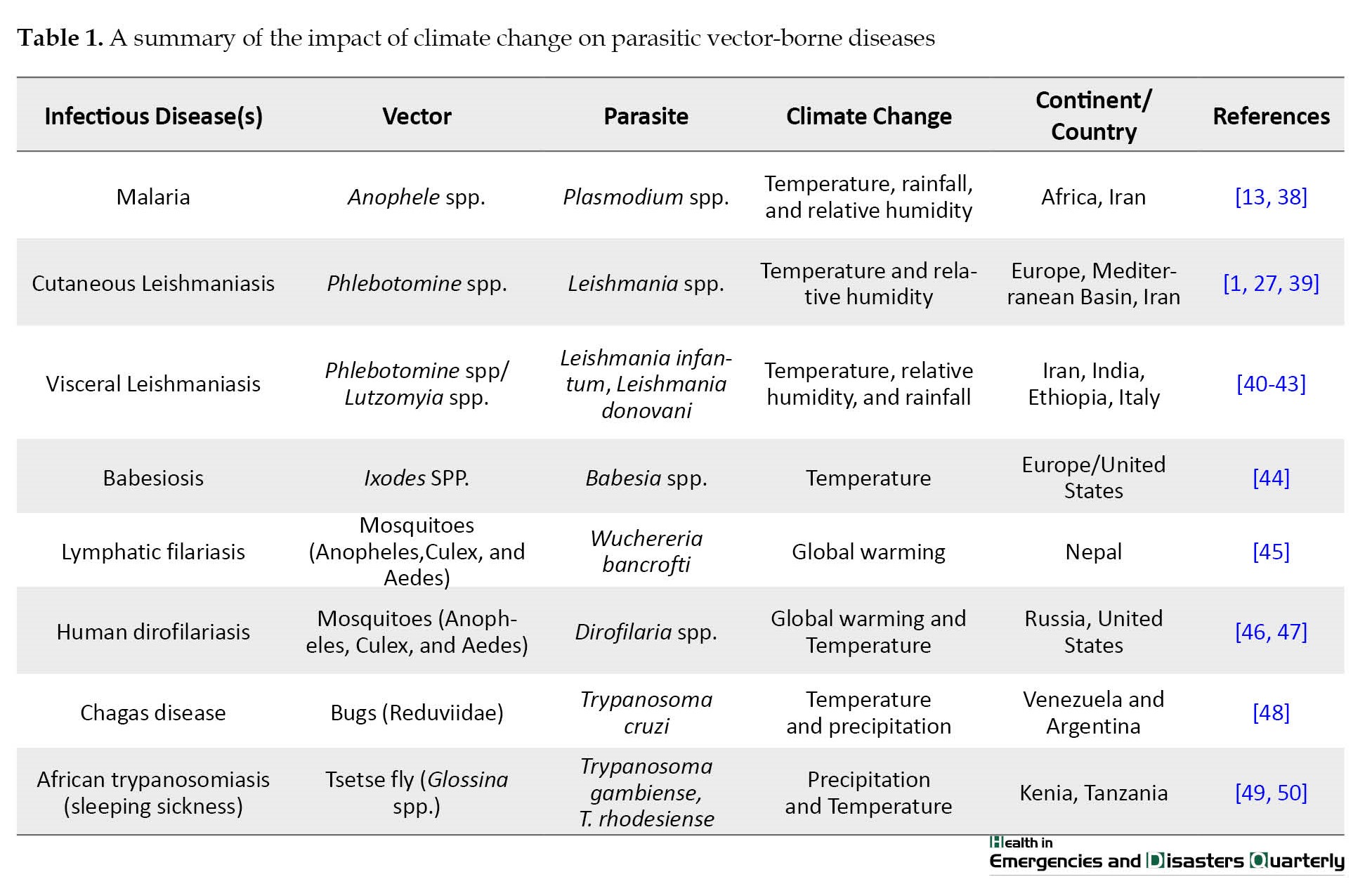
Following, the impact of each of the weather and environmental factors on vector-borne diseases are separately mentioned:
Temperature: Temperature changes may lead to limitations in the distribution and dispersion of disease vectors. The Aedes aegypti mosquito can be mentioned as the vector of yellow fever and dengue viruses [
16]. Experiments show when the water temperature exceeds 34°C, the larvae of this mosquito die; however, the adult insects begin to die when the air temperature exceeds 40°C. Accordingly, if global warming continues, vectors, such as A. aegypti may disappear from some areas with temperatures above the given threshold [
2]. Similarly, malaria-carrying anopheles, mainly found in areas with temperatures above 16°C, may expand dramatically northward with global warming [
8], and temperature lower than this threshold will probably control malaria.
Amount of rainfall: Changes in rainfall may affect disease vectors. Many infectious vector-borne diseases have a positive relationship with rainfall; the growth of the larvae of some vector mosquitoes is accelerated by increasing rainfall and temperature [
17]. Adult Anopheles mosquitoes, the vector of malaria, grow in the clean water of natural ponds; however, drought may limit the breeding sites of these mosquitoes in terms of quantity and quality, thus leading to decreased vector population and consequently decreased vector disease [
18, 19]. Studies conducted on the population changes of Anopheles mosquitoes in Iran also indicate the more effective role of drought on the spread of malaria vectors compared to global warming [
20, 21]. However, rainfall is not always suitable for vectors. Excessive rainfall may have negative effects on mosquito populations as heavy rains destroy mosquito breeding sites [
22]. Conversely, drought in humid areas may reduce the rate of water flow in small streams and provide more pools of water as a suitable site for breeding mosquitoes [
23]. The primary vector of the West Nile virus is a type of mosquito called Culex, which usually grows underground and in the dirty water of urban sewers and reservoirs. Drought allows decaying organic matter to accumulate in these pools and create favorable conditions for these mosquitoes. Heavy rain washes away these sewers and drains the pools [
24], thereby limiting the spread of the West Nile virus.
Air humidity: Many disease vectors tend to respond more strongly to changes in humidity. For example, relative humidity affects malaria transmission by affecting the mosquito’s activity and survival. If the average monthly relative humidity is below 60%, the life span of the mosquito of malaria vector will be too short to sustain malaria transmission [
2]. When warm and humid weather replaces dry weather, vector mosquitoes carrying West Nile virus and Lyme disease may be transmitted to unusual regions, such as Canada and Scandinavia [
25]. Low humidity can negatively affect the survival of adult A. aegypti; therefore, it reduces dengue transmission [
2]. Low humidity, especially when accompanied by high temperatures, creates unfavorable conditions for ticks and fleas (e.g. grasslands and forests) and the spread of related infectious diseases is limited [
26].
In some regions of Southern Europe, the risk of disease transmission decreases where the weather conditions become extremely hot and dry. Forecasts show that the climate in Central Europe will be increasingly favorable for the Phelobotomus spp., and the expansion of these favorable climatic conditions for the Phelobotomus spp. will lead to an increased risk of leishmaniasis, even though this dispersion can be controlled to some extent because of the movement ability and limited flight of the mosquito [
1]. Since changes in temperature and relative humidity affect the survival and reproduction of disease-carrying mosquitoes, the intensity and time pattern of the activity of these vectors may change [
27].
Wind: The wind has a double effect on disease vectors. For example, wind may have both positive and negative effects on the life cycle of malaria. Accordingly, strong wind may reduce the possibility of mosquito bites, while simultaneously increasing their flight distance. During the monsoon season, the winds can change the spatial distribution of mosquitoes [
2].
Sunlight radiation: Sunlight may have a synergistic effect on disease vectors. A time series analysis of cholera cases showed that increasing temperature and long-term sunlight have a positive effect on the monthly incidence of this disease [
28]. In particular, high temperature with moderate sunlight provides the most favorable conditions for the outbreak of cholera. However, a relatively low temperature is suitable for the vector of cholera if it is accompanied by long sunlight [
2].
Climate change and disease transmission
Disease vectors are indirectly involved in the transmission of pathogenic agents to humans in terms of ways of disease transmission. Despite the lack of information on the mechanism of climate effect on disease transmission, many studies have proven that climatic variables and weather conditions may affect disease transmission. Temperature change alone or with alterations in other variables, such as rainfall, may change disease transmission. Studies have reported the relationship between annual temperature changes and malaria transmission in African highlands [
29]. Also, the incidence of hemorrhagic fever with renal syndrome is closely related to weather factors, such as temperature, rainfall, and humidity [
30].
Climate change can affect the transmission of infectious diseases through changes in human-pathogen and human-vector contact patterns. A time series study on malaria showed that seasonal changes in the prevalence of malaria can mainly be explained by the minimum temperature at the beginning of the transmission season, which corresponds to the months with the most contact between humans and the vector [
29].
Climate changes may affect the transmission season through the effect on the reproduction and lifespan of the vectors. For example, long transmission seasons lead to the integration of vector-borne diseases in the long term and, as a result, lead to an increase in infection. Over time, these transmission areas are developed and as a result, vectors with the ability to move to higher places emerge. In the past decades, the prevalence of malaria has increased and this is partly related to climate change [
31]. Climate change is vital in shaping the activity pattern and behaviors of humans and other hosts, such as seasonal employment, migration, and winter and summer lifestyles [
32], which in turn can significantly affect disease transmission patterns. For example, visceral leishmaniasis disease reappeared in the cities of semi-arid regions of Brazil because of the rural-urban migration of farmers [
22].
The influence of climate on changing the sensitivity of the vector host
Disease vectors may survive by sheltering in small-scale environments where a change in ambient temperature is not noticeable. For example, in Jalur, Rajasthan, India, A. aegypti mosquitoes grew in household jars or cemented underground tanks and remained hidden against summer temperatures above 40°C [
33]. Similarly, field observations have recorded live larvae of A. aegypti in ice-covered water [
2]. Also, reports show that these mosquitoes in areas, such as Memphis, USA, where winter temperatures usually reach less than 0°C, are observed [
34].
Extreme weather and disease vectors
Extreme weather events refer to the values of a weather or climate variable that are beyond the threshold limit, i.e. higher (or lower) than the range of observed values, which include extreme events on a global scale, such as El Niño (El Nino) and regional or local meteorological risks, such as drought, heat, or flood. Although these events are rare and occur less than 5% of the time, their frequency and intensity are increasing, representing a critical aspect of global climate change [
35]. They are usually associated with dramatic changes in one or more climate variables and can potentially alter the dynamics of human infectious diseases by affecting pathogens, vectors/hosts, or transmission routes [
2].
In several studies, the effects of extreme weather events and meteorological hazards on vector-borne diseases have been investigated. Studies show that heat waves, storms, droughts, and floods have a direct risk for human infectious diseases [
36].
In addition, extreme weather events create conditions that lead to the outbreak of infectious diseases. For example, heavy rains change insect breeding sites, drive rodents out of their nests, and contaminate water treatment systems. Also, these extreme weather events affect the public health of countries through the occurrence of some infectious diseases. Among these cases, the health effects of the flood can be mentioned that the increase in cases of diseases, such as leptospirosis and enteritis caused by Campylobacter were related to the occurrence of floods in the Czech Republic. Similarly, increased cryptosporidiosis in Britain was also linked to flooding [
11]. However, they are mostly empirical studies and no comprehensive understanding exists of the mechanism of how climate conditions change and how they affect disease patterns. This issue can be a crucial reason for the findings of the relationship between extreme weather events and diseases that are sometimes inconsistent or at other times; they are very local or regional. For example, malaria outbreaks in Ecuador, Bolivia, and Peru were because of the heavy rainfall that accompanied the El Niño event in 1983 [
37].
Discussion
Climate change refers to a long-term change in weather conditions as well as a change in the pattern of weather crises (extreme weather) so that it increases and spreads these weather crises. Currently, the impact of climate change on human societies is observable and its effects are evident in people’s lives. Therefore, some societies suggest measures, such as adaptation and control measures to reduce the damage caused by climate change. Among the harms and dangers caused by climate change, health hazards of people in society that are directly or indirectly affected by climate change can be mentioned [
2].
Accordingly, two scenarios are considered for climate change. One scenario is the impact on the distribution and spread of infectious diseases, which is related to the warming of the air. The other scenario is the change in the outbreak of diseases caused by extreme weather changes and climate crises. Climate mainly affects the range and distribution of infectious diseases, while weather affects the timing and severity of disease outbreaks. Climate change affects disease transmission by changing the geographical range of disease vectors while shortening the incubation period of the pathogenic agent [
11]. The effects of climate change on vector-borne infectious diseases are imposed through the impact on pathogens, hosts/vectors, and disease transmission; accordingly, climate change can affect disease vectors in 3 different ways, namely through a direct effect on the growth, mortality, and reproduction of the vectors, by inducing and creating behavioral changes in the vectors that lead to a change in the transmission pattern, and via changing the sensitivity of the vectors, for example, alterations in the physiology of vector [
12,
51].
Disease vectors need a complex ecosystem for survival and reproduction. For example, increased temperature and rainfall expand favorable habitats for malaria vectors. On the other hand, increased temperature with decreased rainfall creates new habitats for the Leishmaniasis-carrying Phlebotomus species, while decreased humidity of the environment causes the transmission of lymphatic filariasis [
52].
Social and economic factors are also vital in predicting the risk of infectious vector-borne diseases caused by climate change [
5]. Vulnerability of the society to climate change causes the risk of infectious diseases. This can be related to social development, including the expansion and improvement of the health system and existing infrastructure in society. For example, in India, unplanned urbanization has led to the spread of dengue fever in these areas [
53]. In Iran, during the desertification project, which was implemented to prevent the expansion of the desert, by planting trees, suitable conditions were provided for mice to live in these areas, which is one of the reasons for increased cutaneous leishmaniasis in recent years.
Various studies have investigated the impact of climate change on infectious diseases [
11,
12] and the relationship between climate and vector-borne diseases, including bacterial and viral diseases, etc. [
18,
27]. However, in the present study, we tried to emphasize the role of climate change on parasitic vector-borne diseases. Although this study was conducted in a more specific manner unlike other studies that were conducted on a wide range of infectious diseases, one of the limitations of the present study was that the research conducted on the effect of climate and some parasitic diseases was limited.
Conclusion
Many vector-borne diseases are affected by climate and weather conditions due to the multiple animal vectors and reservoirs. This means that in this category of infectious diseases, climate changes can lead to the transmission and durability of vectors as well as the expansion of new habitats.
The prevalence and occurrence of infectious diseases is affected by climate change. Among these diseases, we can mention vector-borne diseases, and especially, parasitic diseases caused by mosquitoes are climate-sensitive diseases. Therefore, to reduce the vulnerability to the effects of climate change, it is essential to increase the awareness and knowledge of human societies in this area and take control and preventive measures. Also, to understand the mechanism of the relationship between climate change and disease transmission, more and wider studies are needed. It is suggested to conduct studies in the field of providing prediction models and temporal-spatial analysis of the impact of climate change on infectious diseases and disease vectors.
Ethical Considerations
Compliance with ethical guidelines
There were no ethical considerations to be considered in this research.
Funding
This research did not receive any grant from funding agencies in the public, commercial, or non-profit sectors.
Authors' contributions
All authors equally contributed to preparing this article.
Conflict of interest
The authors declared no conflict of interest.
References
- Semenza JC, Suk JE. Vector-borne diseases and climate change: A European perspective. FEMS Microbiology Letters. 2018; 365(2). [DOI:10.1093/femsle/fnx244]
- Wu X, Lu Y, Zhou S, Chen L, Xu B. Impact of climate change on human infectious diseases: Empirical evidence and human adaptation. Environment is International. 2016; 86:14-23. [DOI:10.1016/j.envint.2015.09.007] [PMID]
- Liang L, Gong P. Climate change and human infectious diseases: A synthesis of research findings from global and spatio-temporal perspectives. Environment International. 2017; 103:99-108. [DOI:10.1016/j.envint.2017.03.011] [PMID]
- Vardoulakis S, Dimitroulopoulou C, Thornes J, Lai KM, Taylor J, Myers I, et al. Impact of climate change on the domestic indoor environment and associated health risks in the UK. Environment International. 2015; 85:299-313. [DOI:10.1016/j.envint.2015.09.010] [PMID]
- Semenza JC, Menne B. Climate change and infectious diseases in Europe. The Lancet Infectious Diseases. 2009; 9(6):365-75. [DOI:10.1016/S1473-3099(09)70104-5] [PMID]
- Rogers DJ, Randolph SE. Climate change and vector-borne diseases. Advances in Parasitology. 2006; 62:345-81. [DOI:10.1016/S0065-308X(05)62010-6] [PMID]
- Harvell CD, Mitchell CE, Ward JR, Altizer S, Dobson AP, Ostfeld RS, et al. Climate warming and disease risks for terrestrial and marine biota. Science. 2002; 296(5576):2158-62. [DOI:10.1126/science.1063699] [PMID]
- Martin PH, Lefebvre MG. Malaria and climate: Sensitivity of malaria potential transmission to climate. AMBIO A Journal of the Human Environment. 1995; 24(4):200-7. [Link]
- Bosello F, Roson R, Tol RSJ. Economy-wide estimates of the implications of climate change: Human health. Ecological Economics. 2006; 58(3):579-91. [DOI:10.1016/j.ecolecon.2005.07.032]
- Zhou Y, Zhuang J, Yang M, Zhang Z, Wei J, Peng W, et al. Effects of low temperature on the schistosome-transmitting snail Oncomelania hupensis and the implications of global climate change. Molluscan Research. 2010; 30(2):102-8. [Link]
- Bezirtzoglou C, Dekas K, Charvalos E. Climate changes, environment and infection: Facts, scenarios and growing awareness from the public health community within Europe. Anaerobe. 2011; 17(6):337-40. [DOI:10.1016/j.anaerobe.2011.05.016] [PMID]
- Gallana M, Ryser-Degiorgis MP, Wahli T, Segner H. Climate change and infectious diseases of wildlife: Altered interactions between pathogens, vectors and hosts. Current Zoology. 2013; 59(3):427-37. [DOI:10.1093/czoolo/59.3.427]
- Babaie J, Barati M, Azizi M, Ephtekhari A, Sadat SJ. A systematic evidence review of the effect of climate change on malaria in Iran. Journal of Parasitic Diseases. 2018; 42(3):331-40. [DOI:10.1007/s12639-018-1017-8] [PMID]
- Ayubi E, Barati M, Dabbagh Moghaddam A, Reza Khoshdel A. Spatial modeling of cutaneous leishmaniasis in Iranian army units during 2014-2017 using a hierarchical Bayesian method and the spatial scan statistic. Epidemiology and Health. 2018; 40:e2018032. [DOI:10.4178/epih.e2018032] [PMID]
- Pakzad R, Dabbagh-Moghaddam A, Mohebali M, Safiri S, Barati M. Spatio-temporal analysis of cutaneous leishmaniasis using geographic information system among Iranian Army Units and its comparison with the general population of Iran during 2005-2014. Journal of Parasitic Diseases. 2017; 41(4):1114-22. [DOI:10.1007/s12639-017-0944-0] [PMID]
- Epstein PR. Climate change and emerging infectious diseases. Microbes and Infection. 2001; 3(9):747-54. [DOI:10.1016/S1286-4579(01)01429-0] [PMID]
- Hoshen MB, Morse AP. A weather-driven model of malaria transmission. Malaria Journal. 2004; 3:32. [DOI:10.1186/1475-2875-3-32] [PMID]
- Gage KL, Burkot TR, Eisen RJ, Hayes EB. Climate and vectorborne diseases. American Journal of Preventive Medicine. 2008; 35(5):436-50. [DOI:10.1016/j.amepre.2008.08.030] [PMID]
- Barati M, Keshavarz-valian H, Habibi-nokhandan M, Raeisi A, Faraji L, Salahi-moghaddam A. Spatial outline of malaria transmission in Iran. Asian Pacific Journal of Tropical Medicine. 2012; 5(10):789-95. [DOI:10.1016/S1995-7645(12)60145-X] [PMID]
- Salahimoghadam A, KHoshdel A, Barati M, Sedaghat MM. An overview and mapping of malaria and its vectors in Iran. Bimonthly Journal of Hormozgan University of Medical Sciences. 2014; 18(5):428-40. [Link]
- Salahi-Moghaddam A, Khoshdel A, Dalaei H, Pakdad K, Nutifafa GG, Sedaghat MM. Spatial changes in the distribution of malaria vectors during the past 5 decades in Iran. Acta Tropica. 2017; 166:45-53. [DOI:10.1016/j.actatropica.2016.11.001] [PMID]
- World Health Organization. Using climate to predict infectious disease epidemics. Geneva: World Health Organization: 2005. [Link]
- Kovats RS, Bouma MJ, Hajat S, Worrall E, Haines A. El Nino and health. The Lancet. 2003; 362(9394):1481-9. [DOI:10.1016/S0140-6736(03)14695-8] [PMID]
- Epstein PR. West Nile virus and the climate. Journal of Urban Health. 2001; 78(2):367-71. [DOI:10.1093/jurban/78.2.367] [PMID]
- Senior K. Climate change and infectious disease: A dangerous liaison? The Lancet Infectious Diseases. 2008; 8(2):92-3. [DOI:10.1016/S1473-3099(08)70008-2] [PMID]
- Gage KL, Kosoy MY. Natural history of plague: Perspectives from more than a century of research. Annual Review of Entomology. 2005; 50:505-28. [DOI:10.1146/annurev.ento.50.071803.130337] [PMID]
- Negev M, Paz S, Clermont A, Pri-Or NG, Shalom U, Yeger T, et al. Impacts of climate change on vector borne diseases in the Mediterranean basin - implications for preparedness and adaptation policy. International Journal of Environmental Research and Public Health. 2015; 12(6):6745-70. [DOI:10.3390/ijerph120606745] [PMID]
- Islam MS, Sharker MA, Rheman S, Hossain S, Mahmud ZH, Islam MS, et al. Effects of local climate variability on transmission dynamics of cholera in Matlab, Bangladesh. Transactions of the Royal Society of Tropical Medicine and Hygiene. 2009; 103(11):1165-70. [DOI:10.1016/j.trstmh.2009.04.016] [PMID]
- Bouma MJ. Methodological problems and amendments to demonstrate effects of temperature on the epidemiology of malaria. A new perspective on the highland epidemics in Madagascar, 1972-89. Transactions of the Royal Society of Tropical Medicine and Hygiene. 2003; 97(2):133-9. [DOI:10.1016/S0035-9203(03)90099-X] [PMID]
- Xiao H, Tian HY, Gao LD, Liu HN, Duan LS, Basta N, et al. Animal reservoir, natural and socioeconomic variations and the transmission of hemorrhagic fever with renal syndrome in Chenzhou, China, 2006-2010. PLoS Neglected Tropical Diseases. 2014; 8(1):e2615. [DOI:10.1371/journal.pntd.0002615] [PMID]
- Tanser FC, Sharp B, le Sueur D. Potential effect of climate change on malaria transmission in Africa. The Lancet. 2003; 362(9398):1792-8. [DOI:10.1016/S0140-6736(03)14898-2] [PMID]
- Viboud C, Pakdaman K, Boelle PY, Wilson ML, Myers MF, Valleron AJ, et al. Association of influenza epidemics with global climate variability. European Journal of Epidemiology. 2004; 19(11):1055-9. [DOI:10.1007/s10654-004-2450-9] [PMID]
- Tyagi B, Hiriyan J. Breeding of dengue vector aedes aegypti (linnaeus) in Rural Thar Desert, North-western Rajasthan, India. Geneva: World Health Organization: 2004. [Link]
- Reiter P. Climate change and mosquito-borne disease. Environmental Health Perspectives. 2001; 109 Suppl 1:141-61. [DOI:10.1289/ehp.01109s1141] [PMID]
- Lubchenco J, Karl TR. Extreme weather events. Physics Today. 2012; 65(3):31-7. [DOI:10.1063/PT.3.1475]
- Watts N, Adger WN, Agnolucci P, Blackstock J, Byass P, Cai W, et al. Health and climate change: Policy responses to protect public health. The Lancet. 2015; 386(10006):1861-914. [DOI:10.1016/S0140-6736(15)60854-6] [PMID]
- Nicholls N. El nino-southern oscillation and vector-borne disease. The Lancet. 1993; 342(8882):1284-5. [DOI:10.1016/0140-6736(93)92368-4] [PMID]
- Mafwele BJ, Lee JW. Relationships between transmission of malaria in Africa and climate factors. Scientific Reports. 2022; 12(1):14392. [DOI:10.1038/s41598-022-18782-9] [PMID]
- Shirzadi MR, Javanbakht M, Vatandoost H, Jesri N, Saghafipour A, Fouladi-Fard R, et al. Impact of environmental and climate factors on spatial distribution of cutaneous leishmaniasis in northeastern Iran: Utilizing remote sensing. Journal of Arthropod-Borne Diseases. 2020; 14(1):56-67. [DOI:10.18502/jad.v14i1.2704] [PMID]
- Ghatee MA, Sharifi I, Haghdoost AA, Kanannejad Z, Taabody Z, Hatam G, et al. Spatial correlations of population and ecological factors with distribution of visceral leishmaniasis cases in southwestern Iran. Journal of Vector Borne Diseases. 2013; 50(3):179. [PMID]
- Singh NS, Singh DP. A review on major risk factors and current status of visceral leishmaniasis in north India. American Journal of Entomology. 2019; 3(1):6-14. [DOI:10.11648/j.aje.20190301.12]
- Leta S, Dao TH, Mesele F, Alemayehu G. Visceral leishmaniasis in Ethiopia: An evolving disease. PLoS Neglected Tropical Diseases. 2014; 8(9):e3131. [DOI:10.1371/journal.pntd.0003131] [PMID]
- Moirano G, Ellena M, Mercogliano P. Spatio-temporal pattern and meteo-climatic determinants of visceral leishmaniasis in Italy. Tropical Medicine and Infectious Disease. 2022; 7(11):337. [DOI:10.3390/tropicalmed7110337] [PMID]
- Gray JS, Ogden NH. Ticks, human babesiosis and climate change. Pathogens. 2021; 10(11):1430. [DOI:10.3390/pathogens10111430] [PMID]
- Dhimal M, Ahrens B, Kuch U. Climate change and spatiotemporal distributions of vector-borne diseases in Nepal--a systematic synthesis of literature. PloS One. 2015; 10(6):e0129869. [DOI:10.1371/journal.pone.0129869] [PMID]
- Kondrashin AV, Morozova LF, Stepanova EV, Turbabina NA, Maksimova MS. Global climate change and human dirofilariasis in Russia. International Journal of Environmental Research and Public Health. 2022; 19(5):3096. [DOI:10.3390/ijerph19053096] [PMID]
- Boss JD, Sosne G, Tewari A. Ocular dirofilariasis: Ophthalmic implication of climate change on vector-borne parasites. American Journal of Ophthalmology Case Reports. 2017; 7:9-10. [DOI:10.1016/j.ajoc.2017.04.004] [PMID]
- Medone P, Ceccarelli S, Parham PE, Figuera A, Rabinovich JE. The impact of climate change on the geographical distribution of two vectors of Chagas disease: Implications for the force of infection. Philosophical Transactions of the Royal Society B: Biological Sciences. 2015; 370(1665):20130560. [DOI:10.1098/rstb.2013.0560] [PMID]
- Messina JP, Moore NJ, DeVisser MH, McCord PF, Walker ED. Climate change and risk projection: Dynamic spatial models of tsetse and African trypanosomiasis in Kenya. Annals of the Association of American Geographers. 2012; 102(5):1038-48. [DOI:10.1080/00045608.2012.671134] [PMID]
- Nnko HJ, Gwakisa PS, Ngonyoka A, Sindato C, Estes AB. Potential impacts of climate change on geographical distribution of three primary vectors of African Trypanosomiasis in Tanzania’s Maasai Steppe: G. m. morsitans, G. pallidipes and G. swynnertoni. PLoS Neglected Tropical Diseases. 2021; 15(2):e0009081. [DOI:10.1371/journal.pntd.0009081] [PMID]
- Dobson A, Molnar PK, Kutz S. Climate change and Arctic parasites. Trends in Parasitology. 2015; 31(5):181-8. [DOI:10.1016/j.pt.2015.03.006] [PMID]
- Atehmengo N, Idika IK, Shehu A, Ibrahim R. Climate change/global warming and its impacts on parasitology/entomology. The Open Parasitology Journal. 2014; 5(1). [DOI:10.2174/1874421401405010001]
- Shah I, Deshpande GC, Tardeja PN. Outbreak of dengue in Mumbai and predictive markers for dengue shock syndrome. Journal of Tropical Pediatrics. 2004; 50(5):301-5. [DOI:10.1093/tropej/50.5.301] [PMID]